Painting technique is a dauntingly large and rather vague subject to discuss. We could provisionally define ‘technique’ as the skills involved in accomplishing a specific artistic intent. But this leaves the notion of ‘artistic intent’ unexamined (it obviously takes infinitely various forms among artists) and tends to suggest that such an intent is rather imposed on the medium of paint. There is no one prescriptive model of ‘good technique’. One can, however, distinguish a narrower, more practical sense of the term as the knowledge consisting of certain axioms of advice on how to avoid using paint in ways that will produce impermanent or visually ineffective, results. Some of these axioms are given in the section of this site: Dos and Don’ts of Technique.
When artistic instruction was centered on the workshop, questions of aesthetics and soundness of physical procedures were inseparable. But towards the end of the 17th century, workshop training began to be supplanted by that of the Academies, whose curricula were generally confined to drawing in dry media. As Delacroix observed, painting pictures and handling colour was seen by a few academic theorists as involving much intelligence; this was reserved for graphic preparation. This ‘de-skilling’ of the business of applying paint caused damage to artistic formations and was the object of frequent criticism.
As other texts in this website reiterate, oil paint is a suspension in one of several kinds of drying oil, either of a coloured dry pigment or of a coloured liquid or ‘lake’ with an inert powder added to give it body. But in both instances, we are dealing with coloured substances, which seem to possess a particular colour because each absorbs to a varying extent some parts of that spectrum of coloured light we perceive, in total, as ‘colourless’ white light whilst reflecting, to a varying extent, other parts. Newton famously demonstrated that daylight was not one but virtually the whole range of all ‘pure’ colours visible to us by breaking it down with a glass prism. Subsequent physicists have concluded that the range of these ‘spectrum’ colours (red-orange-yellow-green-blue-violet) is, subatomically, a range of frequencies with which the packets of energy we call photons hit the human retina. The red end is the lowest frequency visible to the human eye; the violet end is the highest. For other species that have sensitivity to the infrared and ultra-violet extremes of light invisible to us, the spectrum is of course, quite different in extent.
The spectrum is not, of course, several distinct frequencies but a continuous spread of them. But suppose we select a typical or median point from each of the three general ‘zones’ of these frequencies, one from the red-orange zone, one from the yellow-green, and one from the blue-violet. In that case, we can construct a triad of coloured lights from which, if intermixed, most of the remainder of the spectrum can be reproduced, and if the triad is thoroughly intermixed (known as additive mixing), the result is white light. This triad of coloured lights (and within limits, their frequencies are not strictly fixed) is generally known as the triad of primary additive colours. When each primary is mixed with one other primary, a triad of secondary additive colours results.
The behavior of coloured light is fundamental to colour theory. Still, the colours of the light resulting from the mixing of adjacent primaries of coloured lights suffice to demonstrate to anyone who has used a palette that the behavior of coloured substances like paint is usually quite different. This is because the colour we perceive a given paint to ‘possess’ results from the interaction of light that is generally full-spectrum, with a surface that absorbs some parts of that spectrum and, to a greater or lesser extent, reflects others. In fact, the portion of the spectrum reflected by most coloured substances is far broader than we might in common sense, judge them to have as their actual colour. We are psychologically predisposed to conceptualise and distinguish colours in accordance with ‘colour constancy’, which leads us to assume that the part of the light spectrum which a given substance reflects most of all is its ‘proper colour’. If we did not have such a tendency, we would find the day-to-day recognition of objects much more difficult. But there are many instances where the full spectrum of standard daylight is not present, for example, in a room which has intensely coloured window glass or walls. In these instances, that part of the spectrum of light usually reflected by a given substance might be only weakly present in the light falling into and reflected by the room, and our perception of the colour of that substance changes accordingly. This is the cause of that well-known irritation to clothes-buyers and artists alike: metamerism—the change in the colour of objects determined by change in the colour of the light falling on them. For instance, an intensely red light will be so weak in the yellow-blue spectral zone that a ‘green’ object that reflects that zone most of all will appear dark grey or even black.
The complexity of the reflectance of light by paint surfaces explains why the range of their colours far exceeds those in the light spectrum alone. Brown and red earth colours are substances which only weakly reflect certain wavelengths of the red-orange portion of the spectrum whilst absorbing almost completely the yellow-to-violet remainder. This complexity also explains the richness of paint colour in manipulation. The pattern of reflectance of light across the spectrum by each colour is highly idiosyncratic and can be plotted in graph form.
For example, the reflectance profile of a given red pigment peaks at a certain point in the red zone of the spectrum but only tapers away gently on that portion of the graph covering the adjacent orange zone, whilst the profile of another red peaks at virtually the same axial point but tapers sharply away through the orange zone and then tapers only gently through the green-blue-violet zones. In dry or opaque form, these two might appear indistinguishable. But when these pigments are made into paint and brushed out, their differences are immediately apparent: thinner and more transparent zones will disclose the ancillary ‘taper’ or ‘undertone’ of colour. These differences are also disclosed when each red is mixed with another colour: generally, the orange undertone will be far more conspicuous in the resultant mix than the blue.
Here it could be postulated that the red zone of the light spectrum is at the opposite frequency extreme from the violet, and therefore we cannot talk of the “violet side” of red. In terms of the physics of light, this is true. Still, the greater complexity of light reflectance by substances, not to mention the immense complexity of our perceptual processes, licenses us to organise the spectral colours and the non-spectral in various ways. If we take the linear light spectrum and combine the red extreme to the violet extreme, we can form a circular spectrum of apparently seamless colour transition.
The above illustration was made by Eugene Chevreul, one of the principal 19th-century colour theorists. From the mid-17th century onwards, in the writings of Boyle, Newton, Felibien, and Young, attempts were made to reconcile the discoveries of the physicists concerning light with far older artistic traditions regarding the fundamental or ‘capital’ colours: red, yellow, and (sometimes) blue. It had long been suggested that these three colours were (in theory at least) the pre-mixed basis for all other derived colours. But their behavior, when intermixed, seemed at odds with that of light. What we now understand about reflectance and absorbency of light by surfaces explains this.
The traditional artist’s primaries were an unwitting attempt to make a good selection from the spectrum in a manner anticipating those of physicists. But the broad reflectance of the physical primaries means that each absorbs only about one-third of the spectrum: blue reflects a fair amount of green and absorbs only red; red also reflects blue whilst absorbing green; and yellow reflects green whilst absorbing blue. In an approximate way, these artistic primaries’ reflectances overlapped sufficiently to ‘cover’ the spectrum. The demands of printing from colour photographs forced the precise and standardised specification of these colours as the familiar modern ‘ideal’ printers’ primaries. Pleasingly, the modern standard printers’ primaries are precisely the same as the secondary colours derived from additively mixing each pair of what are generally accepted as the standard light primaries. And if the printers’ primaries are similarly mixed in pairs, the resulting triad of ‘subtractively’ mixed secondary colours is precisely the same as the light primaries. Thus, our systems of predicting light and physical colour behaviour interlock.
Obviously, the primary light colours, considered as selections from the linear spectrum, are shifted far more to one side of that spectrum than their physical colour counterparts. In fact the light spectrum visible to us is so greatly biased to the green-blue-violet side that no predetermined primary light triad can reproduce these frequencies entirely; every selection of lights that covers the other parts adequately will fail to cover these. But despite this range of light being fully visible to us, our sensitivity to colour, as disclosed by our conscious power to discriminate colour difference, is biased to the orange-yellow-green side. The usefulness of any physical colour primary triad, whether of printing ink or paint, lies in its forming a basis for mixing colours that we can judge to be different, and consequently it will be shifted to that side of the light spectrum to which we are most cognitively, as opposed to most physically, responsive.
It is in mixing colours that the difference between the behaviour of light and paint becomes most salient, as a comparison between their respective secondary colours demonstrates. Whereas red-orange and green light together produce a yellow more intense in brightness than each of these lights alone, and green light and violet produce a similarly stronger blue, all mixes employing the physical, paint primaries are less intense than their constituents, and because the physical primaries are themselves shifted towards the zone of the spectrum to which we are optimally responsive, the secondaries follow suit.
To put it broadly, the successive additive mixing of the light primaries results in more intense light; the subtractive mixing of successive physical primaries results in something that absorbs most spectral light—a dull, dark grey verging to black. The reason for this difference in result is that when we mix lights, two or more joining streams of photons obviously result in more photons, but the frequencies at which they are travelling are evened out by this collision such that their resultant frequency is at a median point relative to each on the spectrum of light frequencies. But when we mix paints, ‘peak’ reflectance zone of each paint is no longer as strongly reflective as that zone comprised of the combined overlapping ‘taper’ of subsidiary reflected spectral light both possess. Thus yellow paint and blue paint each reflects a considerable but weaker spectral ‘fringe’ of green, and when they are mixed, it is this fringe that becomes the ‘peak’ reflectance of the blend. Paradoxically, the spectral impurity of paint allows it to be mixed in predictable ways.
When artistic instruction was centred on the workshop, questions of aesthetics and soundness of physical procedures were inseparable. But towards the end of the 17th century workshop training began to be supplanted by that of the Academies, whose curricula were generally confined to drawing in dry media. As Delacroix observed, the business of actually painting pictures and of handling colour was not seen by many academic theorists as involving much intelligence; this was reserved for the graphic preparation. This “de-skilling” of the business of applying paint caused damage to artistic formations, and was the object of frequent criticism.
As other texts in this website reiterate, oil paint is a suspension in one of several kinds of drying oil either of a coloured dry pigment, or of a coloured liquid or “lake” with an inert powder added to give this body. But in both instances we are dealing with coloured substances, which seem to possess a particular colour because each absorbs to a varying extent some parts of that spectrum of coloured light we perceive, in total, as “colourless” white light, whilst reflecting, to a varying extent, other parts. Newton famously demonstrated that daylight was not one but virtually the whole range of all “pure” colours visible to us, by breaking it down with a glass prism. Subsequent physicists have concluded that the range of these “spectrum” colours (red-orange-yellow-green-blue-violet) is, subatomically, a range of frequencies with which the packets of energy we call photons hit the human retina. The red end is the lowest frequency visible to the human eye; the violet end is the highest. For other species which have sensitivity to the infra-red and ultra-violet extremes of light invisible to us, the spectrum is of course quite different in extent.
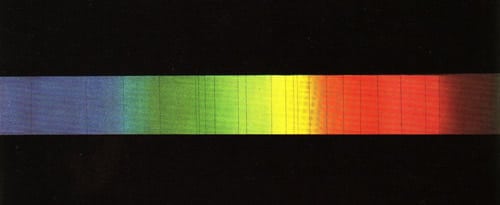
The Linear Spectrum (Chevreul, 1864)
The spectrum is not, of course, several distinct frequencies, but a continuous spread of them. But if we select a typical or median point from each from the three general “zones” of these frequencies, one from the red- orange zone, one from the yellow- green, one from the blue- violet, we can construct a triad of coloured lights from which, if intermixed, most of the remainder of the spectrum can be reproduced, and if the triad is completely intermixed (known as additive mixing), the result is white light. This triad of coloured lights (and within limits their frequencies are not exactly fixed), is generally known as the triad of primary additive colours. When each primary is mixed with one other primary a triad of secondary additive colours results.
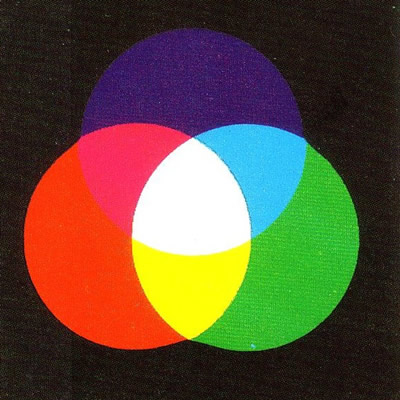
The Triad of Primary Light Colours mixed additively to produce the Secondary Light Triad.
The behaviour of coloured light is fundamental to colour theory, but the colours of the light resulting from the mixing of adjacent primaries of coloured lights suffices to demonstrate, to anyone who has used a palette, that the behaviour of coloured substances like paint is usually quite different. This is because the colour we perceive a given paint to “possess”, results from the interaction of light which is generally full- spectrum, with a surface which absorb some parts of that spectrum, and, to a greater or lesser extent, reflects others. In fact the portion of the spectrum reflected by most coloured substances is far wider than we might in commonsense judge them to have as their actual colour. We are psychologically predisposed to conceptualise and distinguish colours in accordance with “colour constancy”, which leads us to assume that the part of the light spectrum which a given substance reflects most of all is its “proper colour”. If we did not have such a tendency we would find the day-to-day recognition of objects much more difficult. But there are many instances where the full spectrum of standard daylight is not present, for example, in a room which has strongly coloured window glass or walls. In these instances that part of the spectrum of light normally reflected by a given substance might be only weakly present in the light falling into and reflected by the room, and our perception of the colour of that substance changes accordingly. This is the cause of that well known irritation to clothes-buyers and artists alike: metamerism—the change in the colour of objects determined by change in the colour of the light falling on them. For instance, a strongly red light will be so weak in the yellow-blue spectral zone that a “green” object which reflects that zone most of all will appear dark grey or even black.
The complexity of the reflectance of light by paint surfaces explains why the range of their colours far exceeds those in the light spectrum alone. Brown and red earth colours are substances which only weakly reflect certain wavelengths of the red-orange portion of the spectrum whilst absorbing almost completely the yellow-to-violet remainder. This complexity also explains the richness of paint colour in manipulation. The pattern of reflectance of light across the spectrum by each colour is highly idiosynchratic, and can be plotted in graph form.
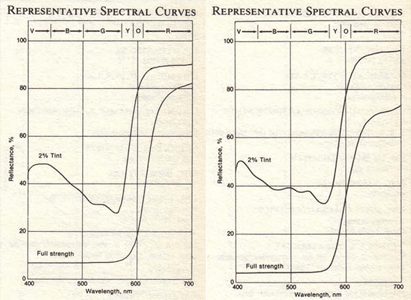
Sample graphs plotting Colour Reflectance for two different red pigments.
For example, the reflectance profile of a given red pigment peaks at a certain point in the red zone of the spectrum but only tapers away gently on that portion of the graph covering the adjacent orange zone, whilst the profile of another red peaks at virtually the same axial point, but tapers sharply away through the orange zone, and then tapers only gently through the green-blue-violet zones. In dry or opaque form these two might appear indistinguishable. But when these pigments are made into paint and brushed out, their differences are immediately apparent: thinner and more transparent zones will disclose the ancillary “taper” or “undertone” of colour. These differences are also disclosed when each red is mixed with another colour: generally the orange undertone will be far more conspicuous in the resultant mix than the blue.
Here it could be objected that the red zone of the light spectrum is at the opposite frequency extreme from the violet, and therefore we cannot talk of the “violet side” of red. In terms of the physics of light this is true, but the greater complexity of light reflectance by substances, not to speak of the immense complexity of our perceptual processes, licenses us to organize the spectral colours as well as the non-spectral in a variety of different ways. If we take the linear light spectrum and simply conjoin the red extreme to the violet extreme, we can form a circular spectrum of apparently seamless colour transition.
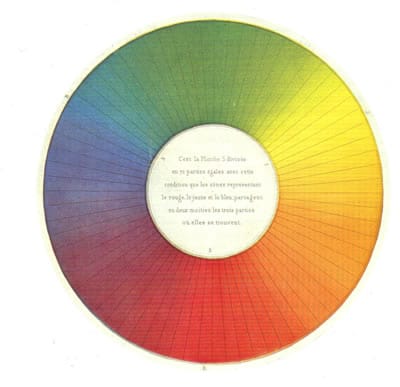
The Light Spectrum represented as a circle.(Chevreul, 1864)
The above illustration was made by Eugene Chevreul, one of the principal 19th century colour theorists. From the mid 17th century onwards, in the writings of Boyle, Newton, Felibien and Young, attempts were made to reconcile the discoveries of the physicists concerning light with far older artistic traditions regarding the fundamental or “capital” colours: red, yellow and (sometimes) blue. It had long been suggested that these three colours were (in theory at least) the pre-mixed basis for all other derived colours. But their behaviour, when intermixed, seemed at odds with that of light. What we now understand about reflectance and absorbency of light by surfaces explains this. The traditional artist’s primaries were in fact an unwitting attempt to make a sufficient selection from the spectrum, in a manner anticipating those of physicists. But the wide reflectance of the physical primaries means that each absorbs only about one third of the spectrum: blue reflects a fair amount of green and absorbs only red, red also reflects blue whilst absorbing green, and yellow reflects green whilst absorbing blue. In an approximate way these artistic primaries’ reflectances overlapped sufficiently to “cover” the spectrum. The demands of printing from colour photographs forced the precise and standardized specification of these colours as the familiar modern “ideal” printers’ primaries. The modern standard printers’ primaries pleasingly happen to be exactly the same as the secondary colours derived from additively mixing each pair of what are generally accepted as the standard light primaries. And if the printers’ primaries are similarly mixed in pairs the resulting triad of “subtractively” mixed secondary colours is exactly the same as the light primaries. Thus our systems of predicting light and physical colour behaviour interlock.
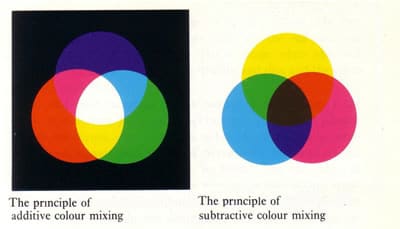
The Triad of Primary Lights and the Triad of Printers’ Primary Colours compared.
Obviously the primary light colours, considered as selections from the linear spectrum, are shifted far more to one side of that spectrum than their physical colour counterparts. In fact the light spectrum visible to us is so greatly biased to the green-blue-violet side that no predetermined primary light triad can reproduce these frequencies entirely; every selection of lights which covers the other parts adequately will fail to cover these. But despite this range of light being fully visible to us, our sensitivity to colour, as disclosed by our conscious power to discriminate colour difference, is biased to the orange-yellow-green side. The usefulness of any physical colour primary triad, whether of printing ink or paint, lies in its forming a basis for mixing colours which we can judge to be different, and consequently it will be shifted to that side of the light spectrum to which we are most cognitively, as opposed to most physically, responsive.
It is in mixing colours that the difference between the behaviour of light and paint becomes most salient, as a comparison between their respective secondary colours demonstrates. Whereas red-orange and green light together produce a yellow more intense in brightness than each of these lights alone, and green light and violet produce a similarly stronger blue, all mixes employing the physical, paint primaries are less intense than their constituents, and because the physical primaries are themselves shifted towards the zone of the spectrum to which we are optimally responsive, the secondaries follow suit.
To put it broadly, the successive additive mixing of the light primaries results in more intense light; the subtractive mixing of successive physical primaries results in something which absorbs most spectral light- a dull dark grey, verging to black. The reason for this difference in result is that when we mix lights two or more joining streams of photons obviously result in more photons, but the frequencies at which they are travelling are evened out by this collision such that their resultant frequency is at a median point relative to each on the spectrum of light frequencies, but when we mix paints “peak” reflectance zone of each paint is no longer as strongly reflective as that zone comprised of the combined overlapping “taper” of subsidiary reflected spectral light which both possess. Thus yellow paint and blue paint each reflects a considerable but weaker spectral “fringe” of green, and when they are mixed it is this fringe which becomes the “peak” reflectance of the blend. The spectral impurity of paint, paradoxically, allows it to be mixed in predictable ways.